Scientists Capture First-Ever Images of Free-Range Atoms
Physicists at the Massachusetts Institute of Technology (MIT) have captured the first photos ever of “free-range” atoms. The groundbreaking achievement will help scientists visualize quantum phenomena in real-world space.
The new photos and associated research published this week in the scientific journal Physical Review Letters, relied on novel imaging techniques to resolve single, free-range atoms. The team’s “atom-resolved microscopy” system uses a “lattice of light that briefly freezes atoms in their tracks,” and then uses precisely-tuned lasers to rapidly illuminate suspended atoms, which creates a “picture of their positions before the atoms naturally dissipate,” MIT’s press release explains.
The physicists used the new technique to visualize clouds of various types of atoms, including atoms known as “bosons,” which then bunched into a wave form thanks to a quantum phenomenon. The team also observed “fermions” actively pairing in free space.
“We are able to see single atoms in these interesting clouds of atoms and what they are doing in relation to each other, which is beautiful,” says Martin Zwierlein, the Thomas A. Frank Professor of Physics at MIT.
Two other groups using similar imaging techniques reported their results in the new journal issue as well, including a team led by Nobel laureate Wolfgang Ketterle, the John D. MacArthur Professor of Physics at MIT, and a group from École Normale Supérieure in Paris led by Tarik Yefsah. Ketterle’s group looked at enhanced pair correlations among bosons, while Yefsah’s team imaged noninteracting fermions.
It’s easy to imagine how challenging it is to photograph a single atom given its extremely small size. A single atom is about one-tenth of a nanometer in diameter. Or, put another way, one-millionth the thickness of a single human hair.
Scientists have been able to image atoms before, thanks to techniques like absorption imaging, where a laser shines onto an atom cloud and casts shadows onto an image sensor. However, these techniques show the overall structure and shape of a cloud of atoms, rather than an individual atom.
“It’s like seeing a cloud in the sky, but not the individual water molecules that make up the cloud,” Zwierlein says of absorption imaging.
Zwierlein and his colleagues used a much different technique. Atom-resolved microscopy requires a cloud of atoms to be held in space by a laser beam trap. Within this trap, the atoms can still freely interact with each other, which is vital to understanding the quantum phenomena at play. A lattice of light, as mentioned earlier, then freezes the atoms in their positions. Finally, a second laser enters the scene, illuminating the suspended atoms. Their fluorescence reveals their position to a highly specialized sensor.
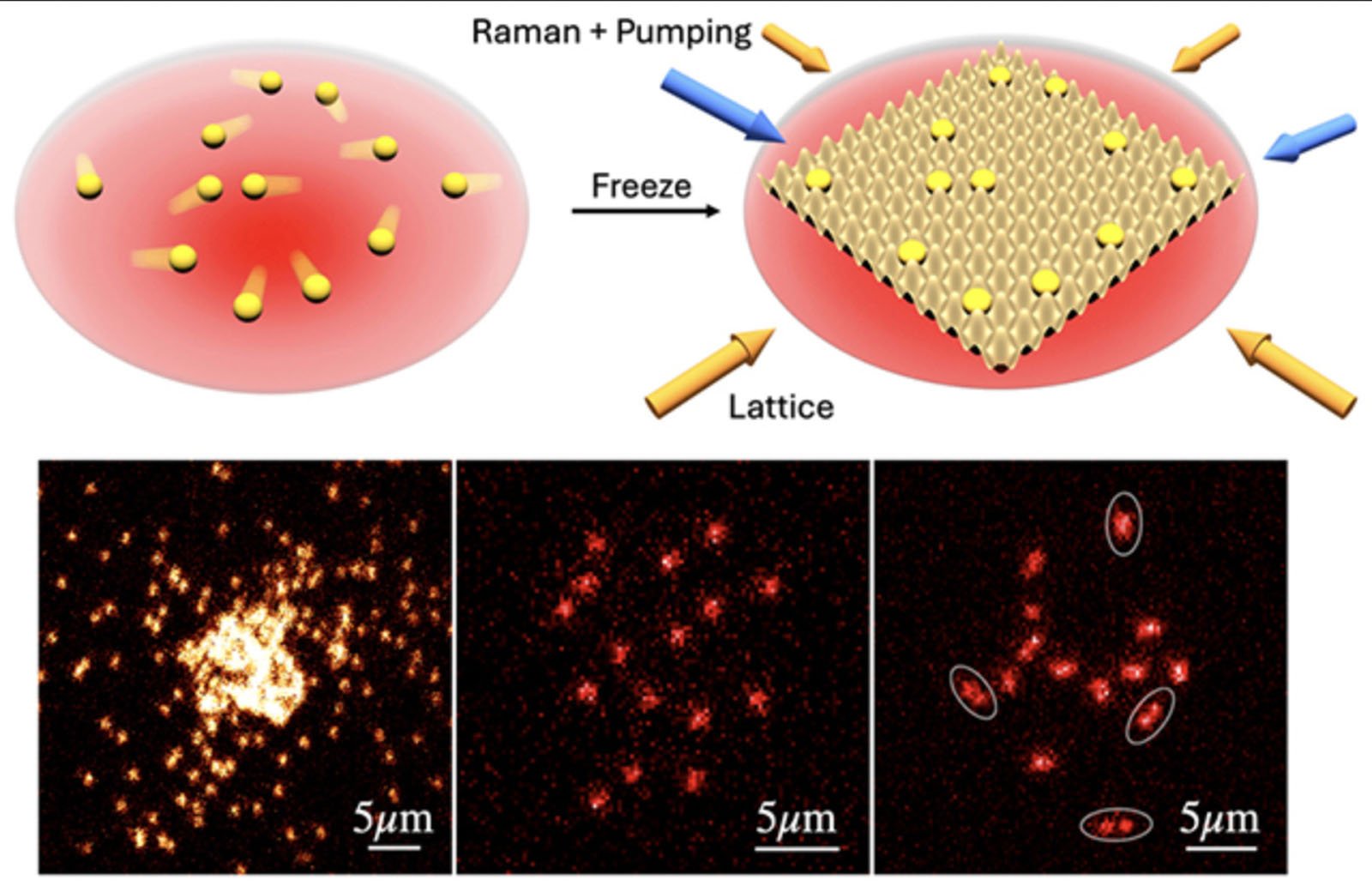
“The hardest part was to gather the light from the atoms without boiling them out of the optical lattice,” Zwierlein says. “You can imagine if you took a flamethrower to these atoms, they would not like that. So, we’ve learned some tricks through the years on how to do this. And it’s the first time we do it in-situ, where we can suddenly freeze the motion of the atoms when they’re strongly interacting, and see them, one after the other. That’s what makes this technique more powerful than what was done before.”
The new imaging results have been decades in the making for Professor Ketterle. He won the Nobel Prize in Physics in 2001 as part of a team that was the first to produce a Bose-Einstein condensate of sodium atoms, which is a state of matter where all bosons share a unified quantum state. Ketterle’s team now captured novel images of bosons.
Zwierlein’s group imaged individual sodium atoms within the atom cloud and observed the atoms sharing the same quantum mechanical wave. The wave-like characteristic, named after its discoverer, Louis de Broglie, helped spur the modern age of quantum mechanics.
“We understand so much more about the world from this wave-like nature,” Zwierlein continues. “But it’s really tough to observe these quantum, wave-like effects. However, in our new microscope, we can visualize this wave directly.”
The MIT team, for the first time in situ, witnessed bosons sharing a single quantum, correlated de Broglie wave. The observations showed real, physical world phenomena previously only demonstrated through pure mathematics.
“This kind of pairing is the basis of a mathematical construction people came up with to explain experiments. But when you see pictures like these, it’s showing in a photograph, an object that was discovered in the mathematical world,” explains study co-author Richard J. Fletcher. “So it’s a very nice reminder that physics is about physical things. It’s real.”
They will begin using their new imaging technique to observe even more exotic phenomena, such as “quantum Hall physics,” which are situations when interacting electrons display correlated behaviors while in the presence of a magnetic field.
“That’s where theory gets really hairy — where people start drawing pictures instead of being able to write down a full-fledged theory because they can’t fully solve it,” Zwierlein hopes. “Now we can verify whether these cartoons of quantum Hall states are actually real. Because they are pretty bizarre states.”
Credits: The full research paper is available now in Physical Review Journals. The paper, “Measuring Pair Correlations in Bose and Fermi Gases via Atom-Resolved Microscopy,” was written by Ruixiao Yao, Sungjae Chi, Mingxuan Wang, Richard J. Fletcher, and Martin Zwierlein.